Time-resolved Laser-induced Fluorescence Spectroscopy
Table of contents
Instrumentation and Applications
Introduction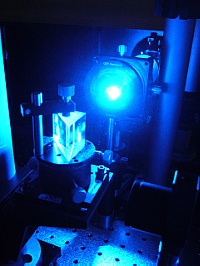
Luminescence spectroscopy is universally applied at IRE to probe the strength, composition, and symmetry of the first coordination shell of multiple actinides and lanthanides The method is particularly valuable due to its trace sensitivity, which means speciations can be determined at environmentally relevant conditions. A wide range of available laser setups and a broad array of sample environments means measurements can be performed in solution for aqueous complexes, in the solid state, e.g. for studying incorporation process or precipitates, as well as in suspension or using wet pastes for the characterization of interfacial species. Experiments can be performed at concentrations down to 10-9 mol/L [for Cm(III)], over a temperature range from 10 – 363 K, and with time-resolution from ~100 fs to 10s of ms.
Background
At IRE luminescence is routinely measured for Am(III), Cm(III), U(IV) and U(VI), as well as Eu(III), and to a lesser extent other lanthanides. The specifics of the observed transitions in excitation and emission will vary with element and oxidation state, but in general three pieces of information can be gathered. Firstly, the emission spectrum itself which will yield information about species contained in the system, their relative composition, and their coordination symmetry. A second piece of information can be obtained from the fluorescence decay constant κ or lifetime τ (= κ-1), which is related to the number and proximity of quenchers in the coordination sphere of the luminescent probe. For Am(III), Cm(III), and Eu(III) the luminescence lifetime can be directly correlated to the number of water molecules surrounding the metal ion using euqations by Horrocks Jr. (Eu) [1], and Kimura (Am, Cm) [2,3] respectively. At low temperatures (T < 20 K) this information can be complemented by excitation spectra which allow for the direct determination of the number of species in a system, as well as their selective excitation to prevent overlaps and obtain single species lifetimes and emission spectra, so called site-selective TRLFS.
Through this wide range of molecular level information combined with trace sensitivity and speciation capabilities, TRLFS has become an invaluable tool for elucidating the chemistry of the actinides in solid, in solution, and at the water/mineral interface.
Instrumentation and Applications
Both, tunable and fixed energy laser system are used for TRLFS experiments at IRE.
- Nd:YAG pumped dye laser systems (Amplitude Continuum, Radiant Dyes) with iCCD detectors, two with closed-cycle liquid-He cryostats (sample temperature > 20 K; Cryophysics)
- Dye laser wavelength can be tuned over wide energy ranges by using a variety of dyes with linewidth ~0.05 cm-1
- Energy output dependy on the dyes conversion efficiency, but is generally in the 5 - 20 mJ/pulse range, with pulse length of 5 - 8 ns, and 20 Hz repetition rate.
- Application: solution studies with Eu(III), Cm(III), direct excitation of Eu and Cm at low temperatures; µTRLFS; polarization-dependent measurements are possible
- Solid state OPO systems with Nd:YAG pump sources with iCCD detectors
- The solid state laser systems can be tuned over much larger spectral ranges without the need for dye changes, with some loss in spectral resolution
- Energy output varies with output wavelength in the 3 - 10 mJ/pulse range for 5 - 10 ns pulses, and 10/20 Hz repetition rate.
- Broad application range for Cm, Eu, other lanthanides, and U(IV)
- Nd:YAG systems with fourth-harmonic generation produce fixed wavelength laser light at 266 nm which can be used for the resonant excitation of U(VI)O22+
- Application: Solution and suspension measurements of U(VI)
- Solid state OPO system with iCCD detector for the production of very short pulses in the ~100 ps range with 1 kHz repetition rate.
- Application: short lived fluorescence, especially Am(III), U(IV), as well as organic fluorophors
The current technical status can be queried here (internal use only).
TRLFS with spatial resolution: µTRLFS
A new experiment has recently become available that allows scanning a sample through the focal point of a focused laser beam. In combination with selective collection of the fluorescence emission this allows to scan a sample with a spatial resolution of ~30 µm, without loss of the speciation capacities of the method. This means that single grains of natural rocks can be characterized separately, instead of investigating each as a single phase system.
An introduction is given in our recent publication on the spatially-resolved speciation of Eu(III) in contact with a natural granitic rock from Eibenstock, Germany.
Example of a µTRLFS distribution map. |
Image: Konrad Molodtsov |
Select results
a) Incorporation of Eu(III) in LnPO4 [5]
Site-selective TRLFS with Eu(III) as luminescent probe was employed to characterize the incorporation of Eu(III) into a series of lanthanide phosphates on the molecular level. Lanthanide phosphates are both, a promising crystalline phase for the immobilization of specific nuclear waste streams, and an important group of minerals, occurring in two modifications as monazite and xenotime.
Excitation spectra (left) and luminescence decay profiles (right) of Eu(III) incorporated into various monazite ceramics. |
Figure: Nina Maria Huittinen |
A linear shift of the excitation wavelength is observed as a function of the ionic radius of the host cation, which is related to the Ln-O bond distance. The emission spectra show the monoclinic structure of the monazite materials is preserved without significant distortion by the incorporation process; while long lifetimes indicate water-free Eu(III), where no energy transfer to the host is observed.
b) Interaction of Cm(III) with α-amylase [6]
Curium(III) TRLFS was used to study the interaction of Cm(III) with the digestive protein α-amylase, a potentially important factor in the bioavailability of trivalent actinides in the human body.
Spectrophotometric titration of Cm(III) with α-amylase as a function of pH. |
Figure: Astrid Barkleit |
Using spectrophotometric titration as a function of pH as well as ligand-to-metal ratio it was possible to derive a full species distribution over the biologically relevant pH range.
c) U(VI)O22+ hydrolysis analyzed by TRLFS and parallel factor analysis [7]
To delineate the complex system of uranyl hydroxo species the combined information from excitation spectra, emission spectra, and luminescence decay profiles was treated with a parallel factor analysis algorithm.
U(VI) TRLFS analysis with parallel factor analysis (PARAFAC) (left); single component spectra from decomposition (right); derived speciation plot for U(VI) as a function of pH (bottom right) |
Figure: Björn Drobot |
Through this approach a complex mixture of five interrelated species could be characterized, which has led to an improved understanding of the thermodynamics and spectroscopic properties of U(VI)O22+.
Contacts
Dr. Moritz Schmidt | moritz.schmidt@hzdr.de | +49-351-260-3156 | General questions, site-selective TRLFS, Eu, Cm | |
Dr. Robin Steudtner | r.steudtner@hzdr.de | -2895 | General questions, U(VI) spectroscopy | |
Dr. Henry Moll | moll@hzdr.de | -2433 | Cm, Eu TRLFS in solution | |
Dr. Alix Günther | a.guenther@hzdr.de | -2433 | Am(III) TRLFS, organic fluorophors, fs-laser system | |
Dr. Astrid Barkleit | a.barkleit@hzdr.de | -3136 | Lanthanide spectroscopy, U(IV) spectroscopy |
Literature
- Horrocks Jr., W.D. and D.R. Sudnick, Science, 206, 1194 (1979).
- Kimura, T. and G.R. Choppin, Journal of Alloys and Compounds, 213-14, 313 (1994).
- Kimura, T. and Y.U.R. Kato, Journal of Alloys and Compounds, 271-273, 867 (1998).
- Molodtsov, M., S. Schymura, J. Rothe, et al., Scientific Reports, 9, 6287, (2019).
- Huittinen, N., Y. Arinicheva, M. Schmidt, et al., Journal of Colloid and Interface Science, 483, 139 (2016).
- Barkleit, A., A. Heller, A. Ikeda-Ohno, et al., Dalton Transactions, 45, 8724 (2016).
- Drobot, B., R. Steudtner, J. Raff, et al., Chemical Science, 6, 964 (2015).
Additional reading:
- Binnemans, K., Coordination Chemistry Reviews, 295, 1 (2015).
- Edelstein, N.M., R. Klenze, T. Fanghänel, et al., Coordination Chemistry Reviews, 250, 948 (2006).
- Geckeis, H., J. Lützenkirchen, R. Polly, et al., Chemical Reviews, 113, 1016 (2013).